Just like trees, people and stars, galaxies have life cycles. A galaxy is born when enough gas and stars coalesce to form a coherent structure—perhaps it starts as one cloud of gas and slowly gathers mass, or maybe it builds up from the collision of two or more clouds. Either way, once formed, a galaxy spends its lifetime making stars, using its reservoirs of gas to create tiny furnaces where nuclear fusion burns elements to release light and energy. A galaxy deemed “alive” shines strongly in ultraviolet light, a signal of young, bright and hot stars. As those stars age, their light changes from hot and blue to cool and yellow or red. When a galaxy contains mostly yellow and red stars and emits little to no ultraviolet light, we consider it retired, or “red-and-dead.” Eventually, if massive enough, it becomes a spheroidal blob, known as an elliptical galaxy, that will likely never birth a new star again.
All around us in nearby space—within, say, 300 million to 600 million light-years—astronomers see dead or dying elliptical galaxies gathered in great ensembles called galaxy clusters. These clusters hold the fossilized remains of the most massive galaxies ever formed—hundreds to thousands of them slowly dancing around one another, gravitationally bound forever in their permanent graves.
But galaxy clusters present a problem for astronomers. Most clusters seem to have been established by the time the universe was only half of its current age. That means the galaxies within those clusters must have birthed most of the stars they contain early in cosmic history. It appears that these galaxies grew to the size of the Milky Way or larger but up to 10 billion years more quickly. The young galaxy clusters, called protoclusters, where these galaxies formed must have been incredibly violent and active places, full of galaxies producing stars at a furious pace. Our current understanding of physics cannot quite explain how they could have grown so big so quickly.
Only recently have astronomers had the telescopic tools necessary to find protoclusters, which are very distant (their light often travels 10 billion years or more to reach us) and frequently hide their most massive galaxy members behind dust. In the past few years scientists have discovered two protoclusters that are providing an unprecedented window into cluster growth. Follow-up observations have revealed that they are, in fact, active and huge—so huge that they challenge our understanding of galaxy formation. If we can solve the riddle posed by galaxy clusters, we may redefine our understanding of the evolution of the universe.
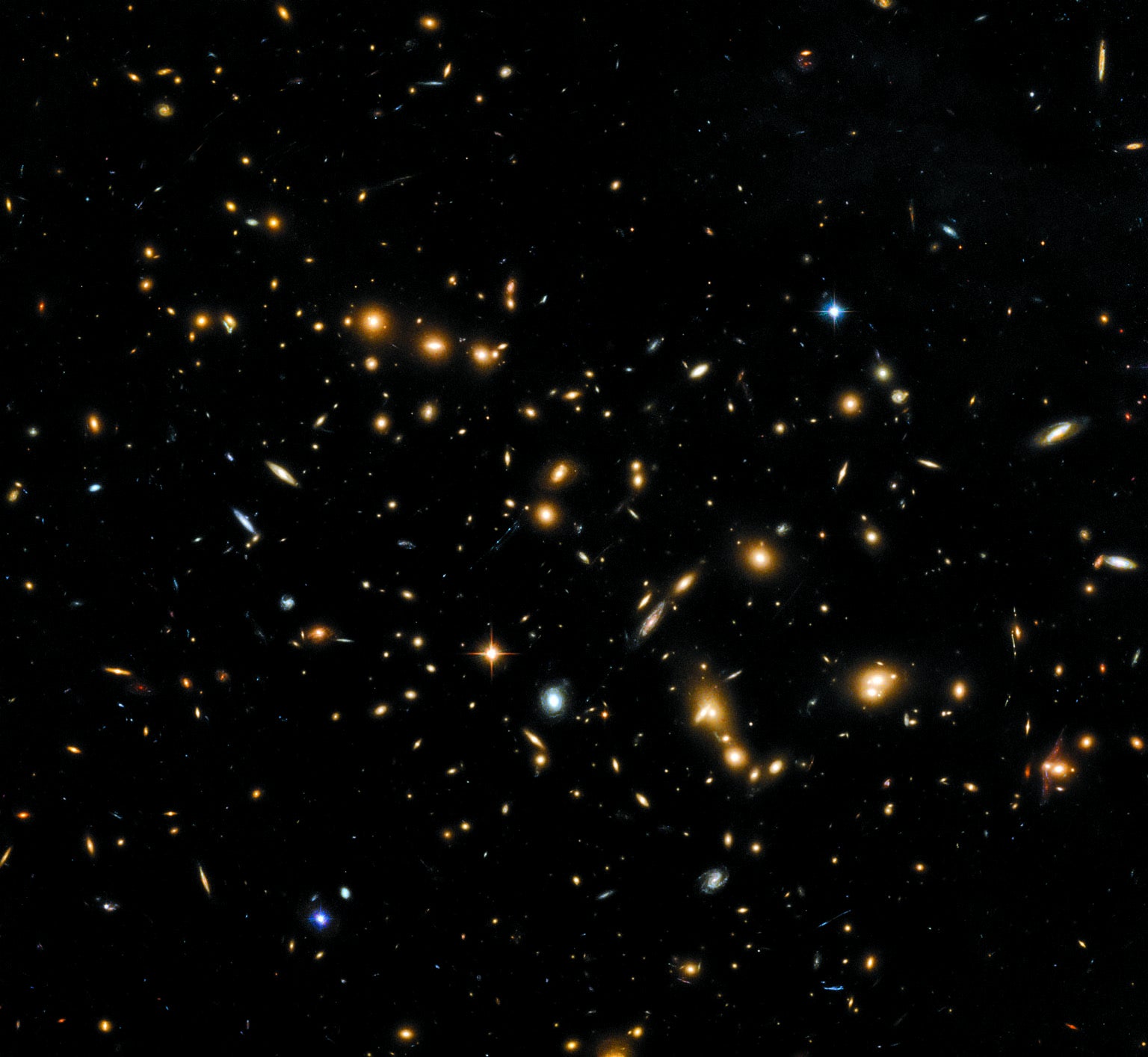
Hunting for Starbursts
The most common type of star-forming galaxy produces roughly one to tens of suns' worth of stars a year. These are often called normal star-forming galaxies. The Milky Way is in this class. Normal star-forming galaxies are metaphorical tortoises, forming stars slowly and steadily over the course of 10 billion years or so, remaining blue and disklike and depleting their reservoirs of fresh gas (fuel for new stars) at a leisurely pace.
Galaxies that produce hundreds to thousands of stars every year are known as starburst galaxies. These are the hares in galaxy evolution. In perhaps 300 million years at most, these galaxies burst into existence, form as many stars as possible as quickly as possible and, in a cosmic blink of an eye, run out of fuel. Starbursts live fast and die young. Astronomers think that they are the best candidate ancestors for the massive, dead elliptical galaxies we see in clusters today.
It stands to reason that if we looked deep enough into space, we would find protoclusters filled with starburst galaxies—tomorrow's clusters of dead galaxies. Yet finding starburst galaxies in protoclusters has proved challenging. Until recently, most of our methods for spotting clusters were developed to preferentially select dying elliptical galaxies or the hot gas that pervades the space between them. Elliptical galaxies and hot intracluster gas appear at the later stages of galaxy cluster evolution, so we need new methods to find their bluer, more star-forming infant counterparts. To make things more difficult, protoclusters are often spread far apart on the sky because the galaxies have yet to fully coalesce into the dense structures we see today. When our most famous and precise telescopes have cameras that span only the width of a pencil (the Hubble Space Telescope, for instance), it is not surprising that we cannot piece together protocluster puzzle pieces that are spread across the sky at distances more than 100 times greater than our telescope's field of view.
Other methods of searching, such as systematically surveying large swaths of the sky, tend to miss starburst galaxies because they are often obscured by dust. The exceptional stellar growth in starbursts generates an overabundance of heavy metals that are produced in the explosive deaths of stars. Once dispersed into space, heavy elements such as iron, carbon and gold collide to form complex dust molecules that absorb and obscure ultraviolet and optical light. Think of the reddening sun during wildfire season: dust dims hotter, more energetic blue light while letting redder light sneak through. The result is that starburst galaxies are nearly invisible when viewed with optical and ultraviolet telescopes, but they shine like beacons when viewed in the cooler infrared spectrum.
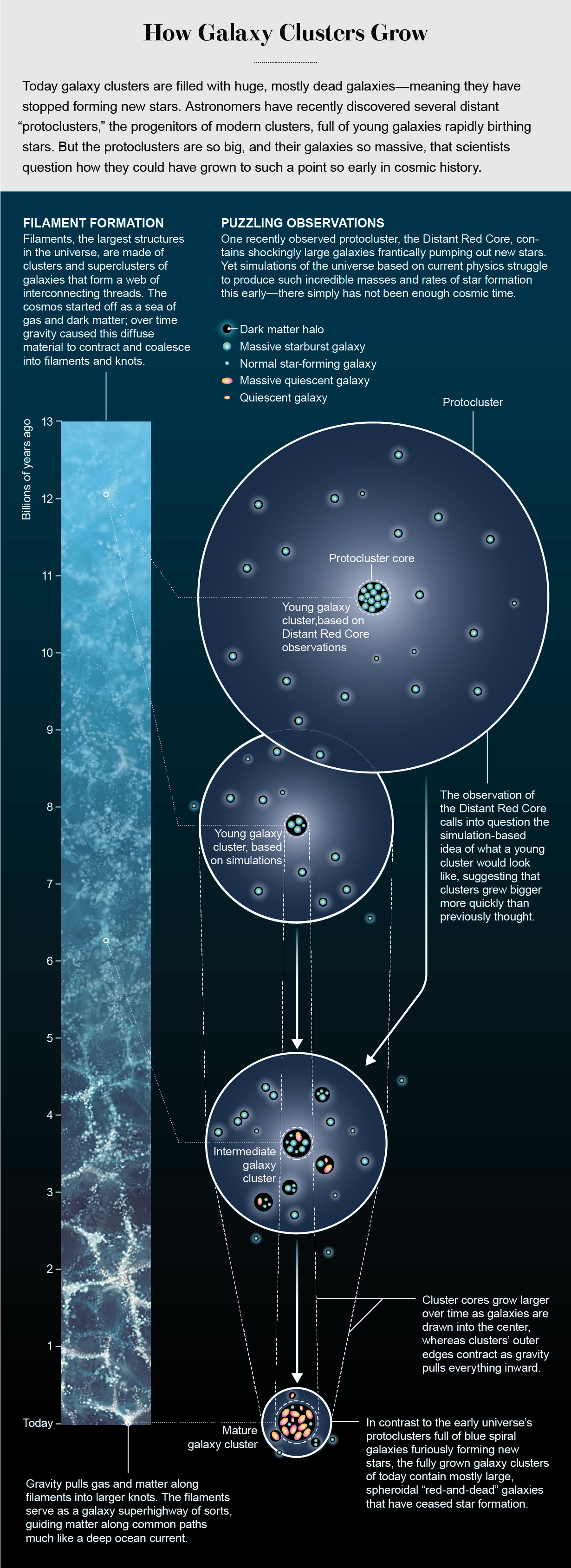
All of this means that until recently, the tools to find and study protoclusters usually missed a key population of galaxies. From the late 1990s through the early 2010s the Submillimeter Common-User Bolometer Array, the Herschel Space Observatory, the South Pole Telescope and the Spitzer Space Telescope revolutionized our understanding of the dust-obscured universe by unveiling millions of galaxies that were previously invisible. Starting about 15 years ago, astronomers began studying the clustering properties of dusty starbursts, and they found that these powerhouses live preferentially near other large and actively star-forming galaxies. But the state of technology was still behind our ambitions; the resolution of infrared and millimeter telescopes was still so low that multiple galaxies would get blended into one large object, even if those galaxies were far apart but lay along the same line of sight. The age of the infrared universe was here, but we needed sharper and more sensitive instruments to fully comprehend what we were seeing.
Finally, in 2013, the Atacama Large Millimeter/submillimeter Array (ALMA) arrived. High in the Chilean desert, this collection of nearly 70 radio dishes works together as a single telescope, reaching resolutions up to 600 times sharper than that of the Herschel telescope. ALMA has transformed many corners of astronomy, including galaxy evolution. (I know several people with tattoos dedicated to this telescope.) The observatory is excellent at detecting dusty, gaseous stellar nurseries throughout star-forming galaxies. With it, astronomers have discovered systems that are both shocking and exciting.
Surprising Behemoths
In 2018 two separate teams of astronomers used ALMA to study the brightest infrared objects they could find in the distant universe. Each team discovered a different conglomeration of dusty, starbursting galaxies that were previously blended together, hiding as one in surveys taken by the first generation of infrared telescopes. SPT2349-56, a group of 14 galaxies, and the Distant Red Core (DRC), a group of 10 galaxies, were both found growing and thriving in different corners of the universe, when the cosmos was only 10 percent of its current age. We see both these budding protoclusters undergoing extreme bursts of star formation—each group birthing nearly 10,000 times as much stellar mass a year as the Milky Way does—across volumes only half the size of our Local Group (which includes our own galaxy plus Andromeda and several smaller galaxies). Estimates of the protoclusters' individual gas reservoirs tell us that if these galaxies continued to form stars at such excessive rates, they would exhaust their fuel supply in just a few hundred million years and become the massive red-and-dead elliptical galaxies that are ubiquitous in fully grown clusters. Moreover, they would complete this cycle well before the present era.
The discovery of these two dust-obscured protocluster cores presented a promising new lens for studying cluster growth, but we were still missing an important part of the picture. The best way to “weigh” a galaxy is to measure the light from its adult star population, which requires data from across the electromagnetic spectrum. But until recently, all observations of protoclusters living in the first two billion years of the universe had been conducted within a narrow energy spectrum (in either the optical or the infrared). Then, in September 2018, my colleagues and I were able to observe, for the first time, ultraviolet and optical emission from a dusty, starbursting protocluster as seen 12 billion years ago: the Distant Red Core. Using the Hubble Space Telescope, the Gemini Observatory and the Spitzer Space Telescope, we captured the multiwavelength perspective necessary to more deeply understand this structure's past and future.
Waiting for Hubble Space Telescope data can be anticlimactic. You know the day that your patch of the sky is scheduled for observation, but you have no idea when you will actually receive your data: you just have to wait for an e-mail notification to tell you to check the archive. The day our protocluster observations were scheduled, I checked my e-mail what felt like every two minutes. I was disappointed when it was time to go to bed and nothing had arrived in my in-box.
The following morning, against my partner's protests, I rolled out of bed immediately and went straight to my computer to see if the data had at last come in. Fortunately, it had been delivered a few hours past midnight. I commenced the download, dancing impatiently like a child waiting for her turn to unwrap presents. Finally, I opened the image. No words can describe how it feels to be the first person to glimpse a part of the universe that no one else has seen. I felt compelled to take a moment to inspect each star and galaxy in the field, to acknowledge their existence. Eventually I snapped back to myself and zoomed in on the part of the sky I was interested in. I saw something remarkable.
This little region of space is violent. At least half of the galaxies there were so messily shaped they must have recently crashed into other nearby galaxies or were still in the process of doing so. When we measured the population of adult stars in these galaxies, we found something incredible—so incredible that it may pose a problem for our current understanding of the universe. Already at this early era, some of the Distant Red Core galaxies had formed three times more stars than our own Milky Way has—but in just a fraction of the time. Yet simulations of the universe based on known physics struggle to produce galaxies this massive so early on. This incongruity between simulation and observation exacerbates a problem that we have known about since the discovery of dusty, star-forming galaxies. Modeling the extreme pace and density of star formation seen in starbursts is difficult because physics predicts the simulated galaxies should either shred themselves apart or heat up so much that they blow out all of the fresh gas needed to grow large enough to match what we see today.
The protocluster as a whole presents another problem: it is shockingly massive. When I first measured it, I could not believe my numbers. I knocked on doors in my department to make sure I was doing the calculations right. Two weeks later I brought the results to a conference to show my collaborators. One said, “There must be a bug in your code.” Another asked, “Are you sure you're not double counting somewhere?” (Turns out there was a small bug in my code, but it was not enough to explain the huge measurement.) Eventually, after double-checking my calculations and trying out different methods, the measurements became undeniable. The Distant Red Core seems to be too big for our universe. We do not know how it could have gotten so large in such a short time.
To better understand its bulk and how much mass was in the form of stars, we zeroed in on the size of the dark matter halo around this protocluster. Dark matter is the most abundant form of matter in any given galaxy and in the universe as a whole. All galaxies and clusters are thought to be surrounded by blobs, or halos, of this mysterious stuff. And although it is invisible and poorly understood, dark matter leaves a clear gravitational signal. There are a variety of ways to infer the amount of dark matter in a given astronomical object, and to cover those methods would require an additional article (or five).
Suffice it to say, we weighed the dark matter component of the Distant Red Core, and according to our simulations, it contains nearly the largest allowable halo mass at that period in the history of the universe. This apparent overabundance of dark matter means that the DRC may be so large that it violates the laws of our universe as we understand them. When we fast-forward our simulations to estimate what the DRC may look like after evolving 12 billion years to the present day, we find that it may grow to be larger than the largest known galaxy cluster, El Gordo. Although we have a healthy margin of error on our dark matter calculation (meaning it could be overestimated), the discrepancy looks even worse when we consider the fact that our observations are capturing only a small percentage of the likely galaxy cluster members; there are probably more galaxies in the DRC that were simply out of the narrow field of view of our telescopes and thus not included in our calculations. This mismatch most likely will grow as we continue surveying and studying this protocluster.
Rethinking the Time Line
Our investigation of the Distant Red Core, along with the discoveries of other potentially similar protoclusters, forces us to reconsider our understanding of galaxy cluster formation. Because the galaxies in clusters are likely to be some of the first galaxies ever, we must determine how such massive objects can form so quickly. Doing so is not just an issue of constraining the physical mechanics and chemistry of star formation inside the first galaxies. It is also a matter of investigating the timing of the conditions that lead dark matter to gravitationally collapse into halos, seeding galaxies. Is it possible that galaxies and structure began forming earlier in the universe than we thought? What does that mean for our understanding of the formation of the first elements? Could these galaxies have forged the right ingredients to build stars with habitable planets around them—and perhaps hosted some of the first forms of life in the universe?
Some of these questions probably will not be answered during my lifetime, but I and other astrophysicists are working hard and fast to address the others. Already we are carrying out more observations of these known protoclusters across the electromagnetic spectrum. We are also developing new methods for identifying large samples of dusty protocluster candidates. With more examples, we may be able to determine whether protoclusters such as the Distant Red Core are examples of a common, yet previously invisible, phase of galaxy evolution that all clusters go through or just rarities. Observers and theorists are forming new collaborations to learn how early in the history of the universe conditions were right for protoclusters akin to those we have discovered—pockets of space overdense with tremendous rates of star formation and outsize masses.
The best way to test our physical models is to look at extremes. In the next few years these colossal congregations of exceptional galaxies will be putting humanity's grasp on the cosmos to the test.