When Judith Klatt began studying the colorful mats of primitive microbes living in a sinkhole at the bottom of Lake Huron, she thought she might learn something about Earth’s early ecosystems. Instead Klatt, a biogeochemist at the Max Planck Institute for Marine Microbiology in Bremen, Germany, wound up confronting one of geology’s greatest unsolved mysteries: How, exactly, did Earth become the only planet known to have an oxygen-rich atmosphere?
Geologic clues suggest microbes may have started releasing oxygen via photosynthesis as early as three billion years ago or even before. But for some reason, it took about half a billion years for that oxygen to build up in the atmosphere and then a billion more for it to reach modern levels and set the stage for complex life. These delays have long puzzled scientists. Some have proposed that chemical reactions consumed much of the gas or that a lack of essential nutrients limited its production.
Now, inspired by the sinkhole work, Klatt and her colleagues have identified another possible explanation, which they describe in Nature Geoscience: early Earth’s days were simply too short.
Soon after the solar system formed, a Mars-sized object crashed into Earth and sent up a spray of debris that became the moon. Drag from our natural satellite has gradually slowed the planet’s rotation ever since, increasing day length from about six hours in Earth’s youth to 24 hours today. Scientists have known about this slowdown for decades and are continuing to refine the details. But few had linked it to oxygen levels—until University of Michigan oceanographer Brian Arbic heard a talk about Klatt’s work with a Lake Huron sinkhole. Arbic, a co-author on the new paper, wondered whether changing day length could have affected photosynthesis over geologic time.
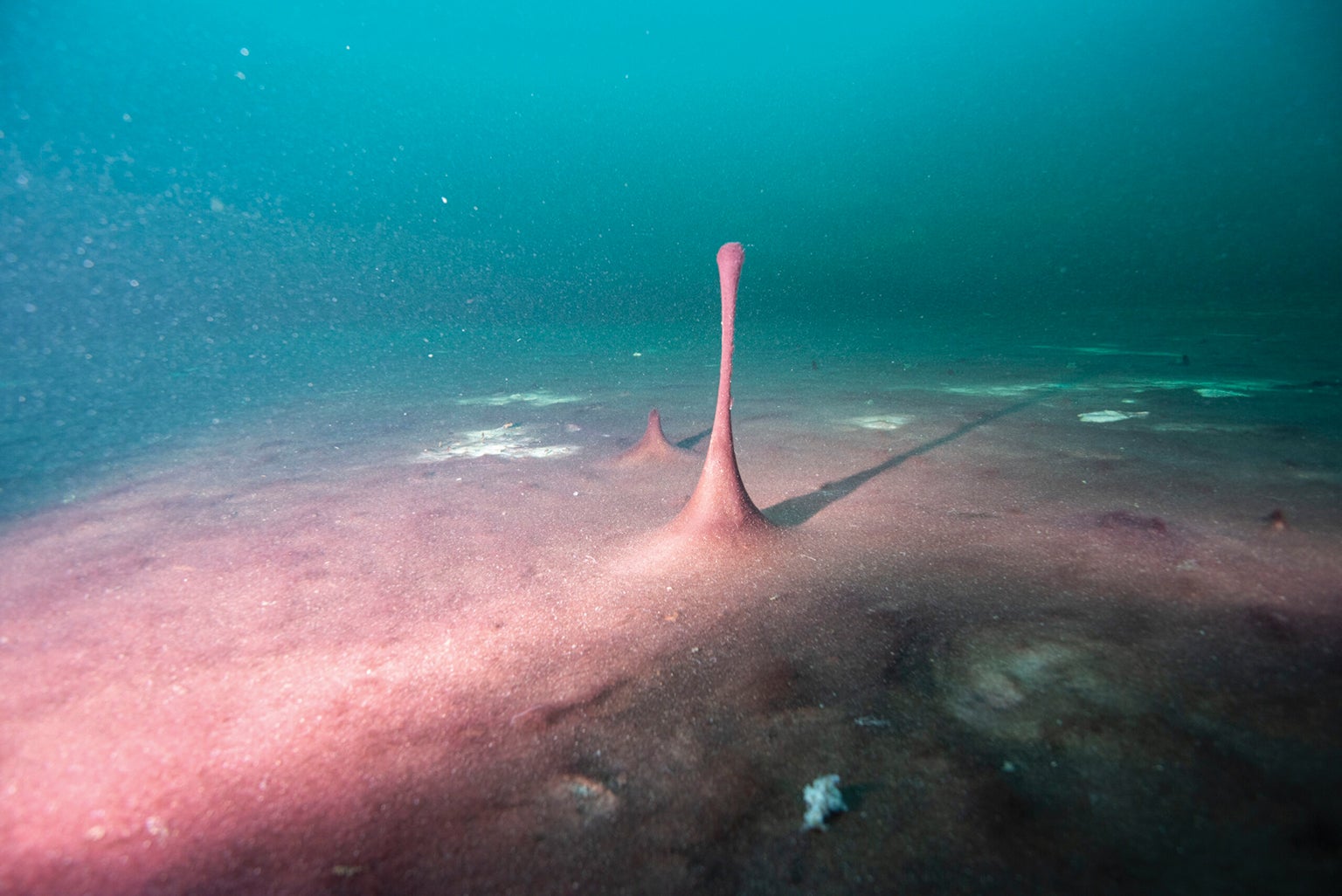
Because it is fed by oxygen-poor, sulfur-rich groundwater, the sinkhole approximates conditions on early Earth, hosting communities of microscopic bacteria that blanket the lake bottom in purple and white mats. Klatt and her colleagues examined how photosynthesizing, oxygen-producing cyanobacteria lie hidden under sulfur-eating competitors at night—and how the two literally swap positions at dawn and dusk. The researchers found that the time they take to trade places creates a lag between when the sun rises and when photosynthesis ramps up, limiting how much oxygen the mats can generate on short days. In fact, Klatt showed in the laboratory that the mats produced no oxygen at all on artificial 12-hour “days” and that oxygen production increased with day lengths beyond 16 hours.
Klatt initially doubted that the sinkhole results could help explain the mystery of the oxygen. “It’s a very special type of community that might not have existed in an ancient Earth,” she says. And without such competition, changes in day length should not matter, because microbes would receive the same total amount of sunlight—just delivered in different increments. But eventually (after thinking for what she calls an “embarrassingly long” time), Klatt realized there was an even more basic link that would apply to any kind of bacterial mat, including those on early Earth: even if oxygen production remained unchanged, longer days would allow more gas to seep into the water—and ultimately into the atmosphere.
That is because the amount of oxygen leaving a mat is limited by how fast gas molecules can diffuse out of it and by how much is consumed by other kinds of bacteria in the mat. Longer days have a drawn-out peak in sunlight, letting more oxygen build up in the mat, which increases diffusion. Critically, longer days also give the gas more time to escape before nightfall, when oxygen-gobbling microbes consume the rest. These mechanisms could have had a strong impact on atmospheric oxygen levels over Earth’s history, the study’s modeling results suggest.
It’s “a simple but elegant idea,” says Timothy Lyons, a biogeochemist at the University of California, Riverside, who was not involved in the study. Lyons says there are still significant unknowns, such as whether early photosynthetic bacteria lived mostly on the seafloor or floated free in the water, where they could release oxygen more easily and without much dependence on diffusion. He suspects that many processes conspired to fill the atmosphere with oxygen—and that day length could certainly have contributed.
Other possible mechanisms include changing emissions of oxygen-consuming volcanic gases, such as hydrogen and methane, and limited supplies of phosphorus, a necessary nutrient for photosynthesis. Benjamin Mills, an Earth evolution modeler at the University of Leeds in England, who was not involved with the study, says he is surprised that scientists had mostly overlooked the role of day length. The challenge now, he says, is assessing “the relative importance of this process versus the other things we know about the global oxygen cycle.”
Both Mills and Purdue University astrobiologist Stephanie Olson, who was also not involved in the study, were impressed by how well the new results match the history of atmospheric oxygenation, including the famous two-step rise and the intervening “boring billion” years—when oxygen levels flat-lined, and day length also stalled at 21 hours. “It’s intriguing that the pattern of oxygen accumulation and the tempo of the slowing of Earth’s rotation rate seem to have occurred in lockstep,” Olson says.
Olson is one of the few others to have proposed a connection between day length and oxygen levels. In a 2020 paper that primarily focused on exoplanets, she described how changes in Earth’s rotation over time might have affected ocean circulation and thus the transport of nutrients such as phosphorus that fuel photosynthesis. Olson and her students are now exploring the idea with computer models. This mechanism and Klatt’s could have worked in concert, Olson says: “I see them as highly complementary, not competing, ideas.”
The thought of a connection between Earth’s rotation and atmospheric oxygen still amazes Arjun Chennu, an ecologist at the Leibniz Center for Tropical Marine Research in Bremen, who co-led the study with Klatt. From the motion of planets to the movement of molecules, he says, “the range of scales at which these interactions have an effect ... is wild.”